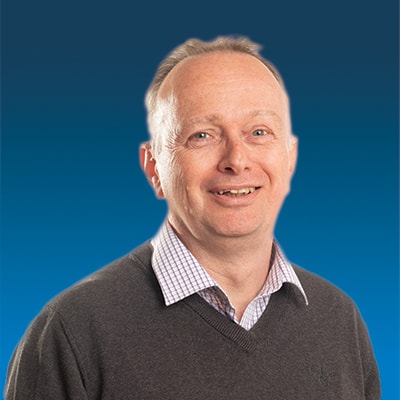
In this series of short technical articles, we look at various topics that illustrate the complexity of renewables as a concept within the ongoing energy transition. Here, PSC’s Richard Adams writes about the impact of asynchronous generation on system protection.
Protection near to renewable sources
Generation background
Traditionally, power networks have been supplied by large synchronous generators, predominantly fuelled by coal, gas or nuclear energy. With the drive for cleaner, greener, and more sustainable energy, the number of synchronous generators in power systems is falling, being replaced by smaller non-synchronous (or asynchronous) connected generation such as solar and wind, along with Battery Energy Storage Systems (BESS).
Large synchronous generators possess significant inertia and provide large fault currents, typically tens of thousands of amps (see Figure 1). In the event of a fault, and for decades, protection engineers have relied upon that fault current to distinguish between normal load currents and faults. In your own home, a fuse or miniature circuit breaker (mcb) relies on current exceeding its threshold to operate and trip your supply in the event of a fault or overload.
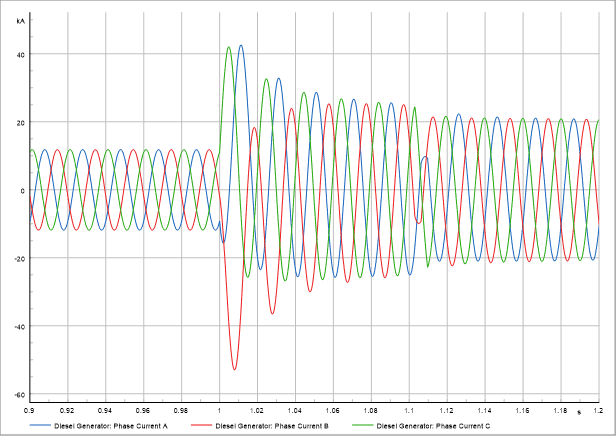
Figure 1: Typical Synchronous generator curve (fault applied at 1 s)
Asynchronous Inverter-Based Resources (IBR) such as solar and wind, on the other hand, do not perform in the predictable fashion of synchronous machines and the proprietary nature of controller design and power electronics physical properties can mean different responses between different manufacturers. In reality, they generally only produce up to around 1.2 times their rated current in the event of a fault, making it much harder to distinguish between a fault and normal loads (see Figure 2). IBRs can be made to produce more fault current, but that comes at a cost as they need more insulation, and it’s not in the manufacturer’s interest to generate higher fault currents.
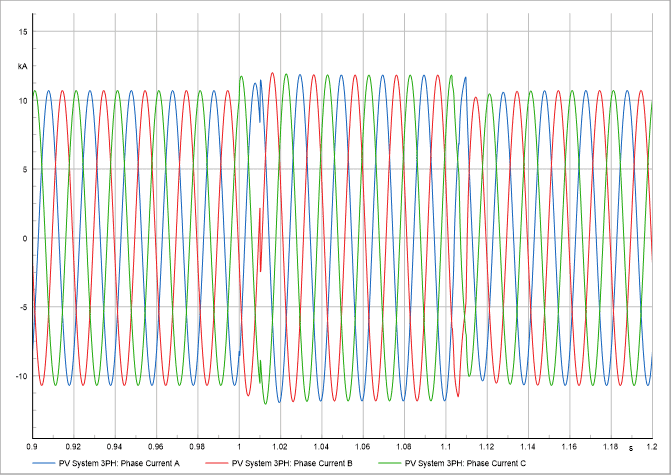
Figure 2 Typical IBR curve (fault applied at 1 s)
The problem
Many suggest that we should find ways to maintain higher fault current levels, using things like synchronous condensers, which would help protection detection techniques. But we should also remember that large fault currents cause significant stress and damage to electrical equipment. The flip side is that lower fault currents are much kinder to the equipment, so protection engineers should find new ways of detecting and distinguishing faults from normal load conditions.
Protection attributes
Under normal conditions, protection should not operate, and may remain like this for many years. In the event it is called upon to act at some point, the protection should display the following attributes:
- Reliability: It must operate when required.
- Selectivity: It should isolate only faulty sections and minimize disruption to healthy circuits.
- Stability: It should have the ability to remain unaffected by conditions external to protected zones, e.g., load currents and external fault conditions.
Reducing the available fault current potentially affects the selectivity of protection and its reliability, neither of which are tolerated when a fault occurs.
Fault detection
Three well-used protection techniques are as follows:
- Overcurrent: The presence of a fault in a system is usually accompanied by an increase in current.
- Impedance: During a fault, the voltage will drop and the current increases – the measured impedance falls.
- Differential: Under normal operation, the current entering a circuit is the same as the current leaving it. Under fault conditions, this is not true.
As mentioned above, the reduction of fault levels makes overcurrent detection difficult, so what about impedance? Unfortunately, distance relays have been shown to be unreliable near to inverter-based sources due to the characteristics and sequence components of the fault current, so their use is not currently recommended [1] [2] [3] [4] [5]. This is at least in part because distance protection utilizes negative sequence current, which is reliable with synchronous generation, but not with IBRs, although it could be possible for IBRs to provide negative sequence current, subject to further research being conducted.
Thankfully, current differential protection still provides a satisfactory protection technique, working, in simplistic terms, on the basis of comparing current entering a zone with current leaving and if there are differences, then it must be due to a fault in that zone.
Recognizing the above issues, protection manufacturers and research institutions have published numerous articles regarding protection of or near to renewable sources, with new distance protection algorithms and alternative techniques suggested. One such new technique [6] [7] now available is protection based on traveling waves, which does not rely on fault current magnitude to establish fault conditions. The technique of using traveling waves to detect a fault and its location is not new, but it’s been mainly found in fault locators until relatively recently, though now relays using such techniques have become commercially available. These use the electromagnetic waves generated during a fault to determine the fault location and can produce a very accurate fault location.
System Protection Schemes (SPS) or Remedial Action Schemes (RAS) as they are sometimes called, are another technique to protect the system, though these do not detect faults as such but rather are configured to detect predetermined system conditions (maybe based on studies) and take pre-emptive action to maintain system stability, voltage and frequency. Such actions could include adjustment or tripping of generation and or load or system reconfiguration.
Final words
For some time now, people have been saying that system inertia and fault levels will drop in the future as more renewable generation is connected, however, we are already seeing some networks, or parts of networks, with very low fault levels due to the amount of inverter-based generation already connected. These issues are no longer for the future; the future is here!
References
[1] | A. Tsylin, R. Kruse-Nielson, G. Yang and B. Sedaghat, “Influence of Collection Network Parameters on Performance of Distance Protection Directional Elements in Offshore Wind Farms,” in DPSP 2020, Liverpool, 2020. |
[2] | C. Brantl, P. Ruffing and R. Puffer, “The Application of Line Protection Relays in High Voltage AC Transmission Grids Considering the Capabilities and Limitations of Connected MMCs,” in DPSP 2020, Liverpool, 2020. |
[3] | J. Wang, Y. Y. Li and F. Hohn, “Impact and Challenges of Conventional Protection Solutions in Wind Farm Connected Grids,” in DPSP 2022, Newcastle Gateshead, 2022. |
[4] | N. George and O. D. Naidu, “Distance Protection Issues with Renewable Power Generators and Possible Solutions,” in DPSP 2022, Newcastle Gateshead, 2022. |
[5] | B. Kasztenny, “Line Distance Protection Near Unconventional Energy Sources,” in DPSP 2022, Newcastle Gateshead, 2022. |
[6] | E. O. Schweitzer, A. Guzmán, M. V. Mynam, V. Skendzic, B. Kasztenny and S. Marx, “A new travelling wave fault locating algorithm for line current differential relays,” in DPSP 2014, Copenhagen, 2014. |
[7] | J. Wang, Y. Li, P. Cost and S. Zubic, “A Novel Pilot Protection for Transmission Lines Based on Full Travelling Wave Signal Polarities,” in DPSP 2020, Liverpool, 2020. |
Series links
The complexity of renewables, Part 1 – PSC Consulting
The complexity of renewables, Part 2 – PSC Consulting
The complexity of renewables, Part 3 – PSC Consulting
The complexity of renewables, Part 4 – PSC Consulting
The complexity of renewables, Part 5 – PSC Consulting
The complexity of renewables, Part 6 – PSC Consulting
The complexity of renewables, Part 7 – PSC Consulting
The complexity of renewables, Part 8 – PSC Consulting
The complexity of renewables, Part 9 – PSC Consulting